Theory, Experiment, and Synthesis
To journey from the Pico meter sizes of atoms to the centimeter or meter sizes of most material applications, I use a variety of spectroscopic and computational techniques. A great challenge of this style of research is connecting the ideal laboratory environment--where we discover most exciting phenomena--with the “messy” environments and cruel practicalities of the real world. To combat this challenge, my work leverages disciplinary diversity and incorporates experimental and theoretical techniques from chemical engineering, materials science, physics, chemistry, computer science, and geology.
Research Methods
- Quantum Chemistry
- Solid-state Nuclear Magnetic Resonance
- Machine Learning
- Scalable Fabrication of Nanomaterials
- Influence though Policy Collaborations
Quantum chemistry
Computational approaches enable both a prediction and possible interpretation of experimental results, in many cases enabling and extending conclusions drawn initially from expensive or difficult to obtain experimental NMR spectra. My theoretical work in Density Functional Theory (DFT) primarily seeks to isolate contributions of density and energy driven errors that add considerable uncertainty to DFT predictions. Computationally, I use DFT for understanding structure, arrangements, and spectra of salts and oxides.
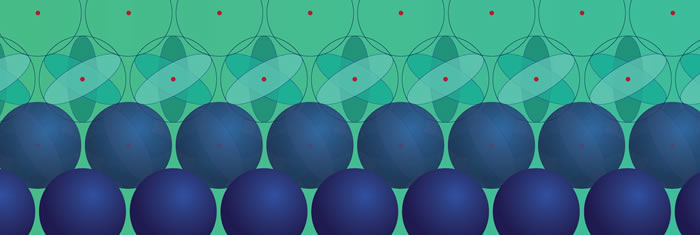
Solid-state Nuclear Magnetic Resonance
Within Solid State NMR, most of my focus is in improving our ability to study multicomponent systems, especially those with impurities. This entails developing both analysis software and more sensitive hardware. Within software, my work seeks to build interactive and easy to use databases for sharing spectra and observations. For hardware, I am developing “tiny lines”, small diameter transmission line probes, with a specific interest in low gamma nuclides.
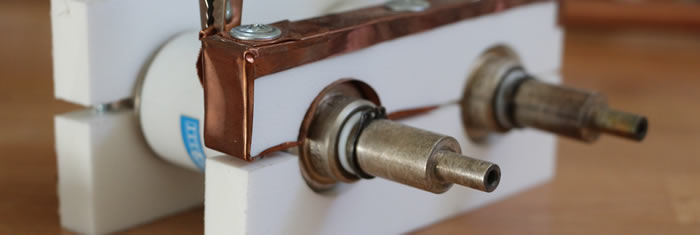
Machine Learning
In sharp contrast to the typical use of machine learning for large-n datasets, machine learning for chemistry and materials typically deals with small datasets with high dimensionality. Modern spectroscopic data relevant to today’s most pressing problems are often highly complex, confusing, and typically difficult to interpret. Component analysis techniques attempt to extract the most meaningful aspects of the data and re-present them to the human in meaningful ways. My work in this domain merges spectroscopy with computer science and mathematical statistics to create more accurate algorithms for understanding chemical data.
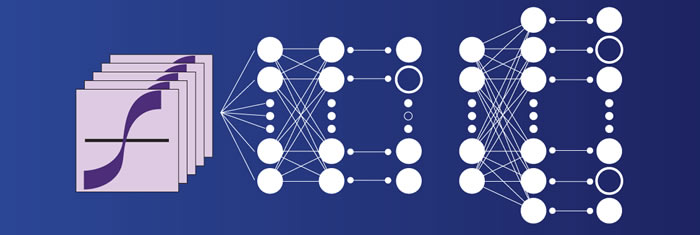
Scalable Fabrication of Nanomaterials
Because nanomaterials have demonstrated some fantastic properties, many forward-looking technologies, like fuel cells, hinge on these materials. However, tiny things are often difficult to make in anything other than tiny amounts, a common problem in scaling up many of these promising technologies. My research efforts in nanomaterials focus on high volume production (for a research setting), with a particular interest in enabling academic researchers to experiment using systems that can be easily translated to industrial settings. Currently, my work is focused on producing inexpensive and adaptable devices for electrospinning an expansive range of materials, solving nanoscale synthesis limitations with novel hardware.
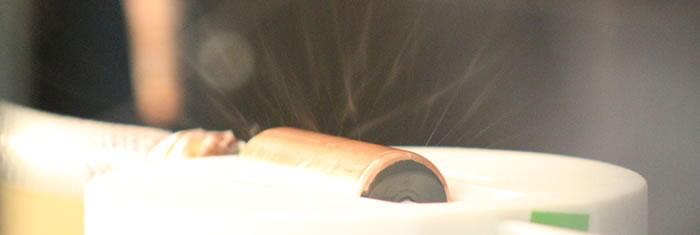
Influence through Policy Collaborations
Science that never leaves the benchtop has little value to society at large. A portion of my work seeks to translate the physical sciences, especially of geochemical systems, to policy-applied scholarly publications by collaborating with environmental and nuclear policy colleagues. While these publications seldom offer novel results, they summarize scientific insight to a audience curious to have the latest research cleanly explained.